Reports
Entropy production in turbulence parameterisations
The idea of M4 is the stochastic or counter-gradient parameterisation of momentum and heat fluxes in forced dissipative systems like the atmosphere and ocean.
Dear Reader,
Yesterday I dropped my beloved teacup. Viewing the broken fragments from the perspective of an enthusiastic tea addict: hope for occurrence of the backward process called self-repair. From the sober viewpoint of a physicist: second law of thermodynamics and entropy.
Macroscopic (isolated) systems evolve in a one-way direction of time, towards states with increased entropy seeming to be in contradiction to the underlying microscopic equations that are needed for their description. For instance, Newton’s law of motion for classical systems is symmetric under time reversal; no preference of a certain time direction; no preference of neither the forward nor backward process. Then why is there a break of time symmetry at macroscopic level (irreversibility)?
The second law of thermodynamics is valid in a statistical sense for large system sizes (average statement in thermodynamic limit) within the frame of equilibrium thermodynamics and can be generalised by the Fluctuation Theorem (FT). Resulting from statistical physics this theorem with its different versions connects microscopic and macroscopic behaviour for time reversal systems of arbitrary size arbitrary far driven out of equilibrium in form of an analytical expression of probability ratio of observing a trajectory of a system (in phase space) to its time reversed counterpart.
The essential quantity of the FT is the dissipation function, an entropy-like quantity in non-equilibrium related to internal entropy production under specific conditions. The latter quantity is especially important in regard to our project M4. Here, ‘our’ consists of the Hamburg part, Richard Blender, Valerio Lucarini (Reading) and me (since October last year), and of the Rostock part (IAP) composed of Almut Gaßmann and Bastian Sommerfeld.
The idea of M4 is the stochastic or counter-gradient parameterisation of momentum and heat fluxes in forced dissipative systems like the atmosphere and ocean. These sub-scale fluxes are related to energy dissipation and backscatter; as well related to positive and negative entropy production. Is it possible to put more physics in these turbulence parameterisation schemes with the usage of the FT? However, it requires the applicability of FT for non-time reversal systems (Navier–Stokes equations). As a representative example for turbulence toy models I use the class of shell models (as a first step) to get a basic notion for the incorporation of the FT with the final aim of modification and improvement of climate prediction models. I am looking forward to this challenge. Thank you for your attention.
Solving equations faster and more accurately
Designing high-order numerical methods needs a good understanding of the mathematical equations we want to solve.
Hi, I’m Claus and I am a PostDoc in Project M5. The focus of my research in this project is the development and analysis of high-order advection methods and high-oder flux evaluation techniques for ocean models. Our goal is to reduce spurious diapycnal mixing in ocean models and I’m working on the intersection between mathematical analysis and numerical methods to help with that.
High-order numerical methods for flow computations are becoming increasingly more popular in computational engineering, but may be not as widespread in climate and ocean science. So what’s the deal with high and low order?
Classical finite differences and finite volume methods for the discretization of partial differential equations use one piece of information in each cell (say, a function value at the cell-center or an integral average over the cell) and put this information into a discrete version of the PDE. This allows for fast and robust algorithms, but in order to resolve small scale features, we often need very fine grids. On the other hand, the high-order methods we are interested in, use higher degree polynomials or other nonlinear functions in each cell. This extra information allow us resolve more features of the solution, even on coarser girds. In many computational fluid dynamics applications this leads to a smaller overall computational time and we would like to show that this is also true for problems in ocean science.
However, carelessly throwing high-order polynomials at your problem is a sure recipe for failure. If we want to include more features of the analytical solution in our numerical solution, we need a good understanding of the analytical properties and how they can be translated into our numerical scheme. For our particular application, e.g., we want tight control over diffusion properties and need to tune our methods to avoid artificial diffusion without losing stability properties that numerical diffusion brings.
In short, designing high-order numerical methods needs a good understanding of the mathematical equations we want to solve. I’m happy to be involved with learning more about the equations in ocean science, so that we can solve them faster and more accurately.
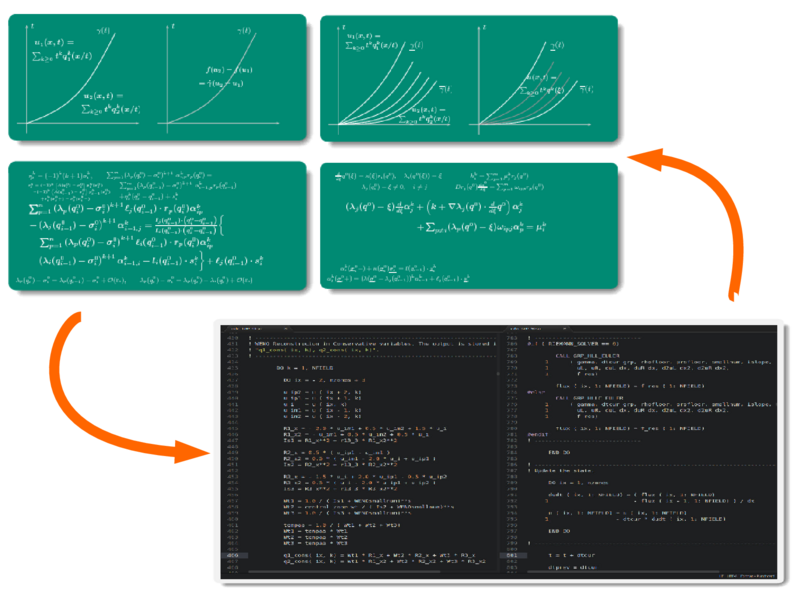
Minimising spurious mixing in numerical ocean models
The possibility for direct application of newly developed model techniques within leading national climate model systems is very stimulating.
Hi. Last month I started working as a postdoctoral researcher in subproject M5. After being involved already in the project's proposal and review process, I am very happy to finally participate in this exciting TRR. As a co-developer of the coastal ocean model GETM, I am strongly interested in the development of energy-consistent modelling techniques. In ocean models the advective transport of water masses is prone to energetic inconsistency. On the discrete model level this transport is associated with truncation errors causing spurious diapycnal mixing, which artificially increases potential energy without any physical sources.
Recently, I developed (together with PI Hans Burchard) a new analysis method that can quantify spurious mixing locally in every single grid cell. In M5 this method will be applied now to assess the new adaptive grid techniques and advanced advection schemes, that will be developed at UHH (PI Armin Iske), AWI (PI Sergey Danilov) and IOW (PI Hans Burchard) in order to reduce spurious mixing. I will be responsible for the development of algorithms that during runtime adapt the discrete model layers to the fluid flow (in order to minimise vertical transports across the layer interfaces), to isopycnals (in order to minimise diapycnal transports along the model layers) as well as to regions where high vertical resolution is needed (in order to minimise truncation errors) in an optimal way.
Furthermore, I will successively implement all schemes and algorithms developed in M5 into GETM to identify promising combinations that should finally be included into FESOM (developed by Sergey Danilov), which is the ocean component of the state-of-the-art climate model system ECHAM6/FESOM. With Sergey Danilov and Armin Iske being also PIs in the synthesis project S2, this possibility for direct application of newly developed model techniques within leading national climate model systems is very stimulating.
In the frame of the TRR I am looking forward for the close collaboration with experienced oceanographers, meteorologists and mathematicians, which offers an optimal academic environment for me as a young scientist. To foster the internal collaboration within M5, I will be first employed at UHH for 1.5 years and afterwards at IOW.
Multi-scale instabilities and energy transfers
We expect to foster the understanding of multi-scale processes that are slow evolving and are usually ‘hidden’ behind the faster dynamics.
Since September 2016, I work as a Post-Doctoral Researcher for the TRR. Previously, I was working as part of the DFG funded project MERCI after finishing my PhD at the International Max-Planch Research School at the Max-Planck-Institute for Meteorology in Hamburg.
My research is mainly focused on the various applications of dynamical system theory to geophysical models of simple to intermediate complexity. In particular, I have applied the theory of Covariant Lyapunov Vectors to a quasi-geostrophic two layer model and studied the connection between the unstable and stable directions to their baroclinic and barotropic energy conversions (Schubert & Lucarini, 2015). This type of analysis also allowed it to illuminate some features of simple blocking like patterns (Schubert & Lucarini, 2016).
For the project M1, I will study the properties of multi-scale instabilities using CLVs. The presence of multi-scale features usually impedes efforts to make good predictions. I will investigate the connection between multi-scale instabilities and energy transfers between atmosphere and ocean using firstly a rather simple quasi geostrophic model of the atmosphere and ocean (MAOOAM). Secondly, my interest lies in exploring the multi-scale properties of linear instabilities in a primitive equation model (PUMA). With these investigations using new tools from dynamical system theory, we expect to foster the understanding of multi-scale processes that are slow evolving and are usually “hidden” behind the faster dynamics.
Lyapunov vectors and the geophysical flow
We hope to better understand the effects of melting glaciers related to global warming on the Gulf Stream within the scope of a small model.
Since August 2016 I am a Ph.D. student at Universität Hamburg. Specifically, I am a member of the research group differential equations and dynamical systems at the department of mathematics. Under supervision of Professor Reiner Lauterbach, Professor Ingenuin Gasser and Professor Valerio Lucarini, together with Dr. Sebastian Schubert, I work on the subproject M1:Instabilities across scales and statistical mechanics of multi-scale GFD systems.
In particular, my forthcoming research is based on the numerics of covariant Lyapunov vectors. These vectors identify directions of asymptotic growth rates to small linear perturbations of orbits in a dynamical system. The theory of covariant Lyapunov vectors provides an extension to the stability analysis of equilibria and to Floquet theory. Hence, they can be used to investigate the stability of more complex objects in, for example, geophysical flows.
Furthermore, I am to investigate the dynamics of a low-dimensional model of the Gulf Stream in the context of bifurcation analysis. With the use of local and global properties such as temperature, specific weight and ocean salinity, we hope to better understand the effects of melting of glaciers related to global warming on the Gulf Stream within the scope of a small model.
Before the project, I studied mathematics at Universität Hamburg and wrote my master's thesis on the dynamics of coupled cell systems this year.
I am happy for the opportunity to gain new experience through this project and to contribute to this research.